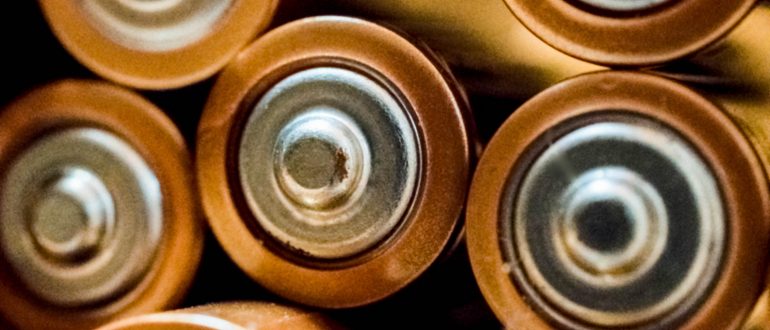
Innovation through technology: The sustainability of lithium batteries in electric vehicles
The production of energy storage systems for electric vehicle applications using lithium-ion batteries form the basis of this bi-regional case study. The assessment draws attention to the sustainability of two stages of the lifecycle of lithium-ion batteries in order to understand resource availability and product sustainability for current and future manufacturing, not only for electric vehicles but also for other electronic devices powered by lithium-ion batteries technology.
Technology is providing new and exciting opportunities to help combat current anthropogenic issues such as climate change. Batteries in particular have become a key element in modern day society; from mobile devices and laptops to the growing markets of clean energy (such as solar panels, wind turbines, etc.) and electric vehicles. With the evolution of the rechargeable battery, society is becoming aware of the benefits it can provide, not only to people, but also to the natural environment.
In response to global efforts to reduce CO2 emissions in the transportation sector, electric vehicles (EVs) have become a growing market.1 It has been estimated that EVs will increase more than thirtyfold by 2030 (see Graph 1), competing against laptops and mobile phones for the use of lithium-ion (Li-ion) batteries.2 The growing interest in the Li-ion battery specifically is due to its long life, lightweight characteristics and its cost-efficiency, which has forecast it to have the highest potential for future energy storage technology.3 The increase in EVs has consequently increased demand for Li-ion batteries, placing a greater pressure on the sourcing of the necessary raw materials such as cobalt, graphite and lithium.4 Worldwide lithium production, for example, has increased by approximately 13% during 2017.5
Although EVs do not directly emit CO2, their overall greenhouse gas emissions depend on the fuel type used to generate the electricity that is used to charge the battery. An EV’s environmental footprint, like any product, also depends on the sustainability of the process of production, including the steps taken to produce and dispose of the battery. One way to analyse its sustainability is to carry out a life cycle analysis.6
Graph 1: Annual global electric vehicle sales are forecast to hit 24.4 million by 2030. Courtesy: Bloomberg Energy Finance
Life Cycle
A product will go through several stages before it reaches the end of its life or “use.” The life cycle of a product has five main stages: Resource Mining, Chemical Production, Product Manufacture, Product Use and Waste Management.7 Companies are becoming more aware of this life cycle and that it is important to analyse how each part of the process is carried out.8 In this particular case, two of the main stages in the life cycle of the Li-ion battery for EVs will be discussed: the resource mining of lithium and the waste management of Li-ion batteries.
The two stages will be based on the following discussions; first, the potential environmental impact of the resource extraction of lithium in Chile and options for improving sustainability, and second, sustainable and efficient waste management in the UK of Li-ion batteries no longer of capacity to power an EV. While the manufacturing stage is just as important in regard to sustainability, focus will be placed upon the direct environmental effects of the life cycle of a Li-ion battery from an EV during the extraction process, and how it is dealt with when it is no longer of use to the consumer.
The salt flats of Chile
Currently, around 70 million cars are produced globally per year.9 Around 7 million lithium ion batteries are manufactured each year, equating to enough batteries for just 10% of vehicle production.10 According to Armand and Tarascon (2008), replacing the world’s 800 million cars and lorries with electric transportation would use 30% of the world’s known reserves of lithium.11 The issue with lithium is not its availability but rather the rate at which it is being produced. 12
The Salar de Atacama in Northern Chile is one of the biggest producers of lithium salt from brine deposits (also known as evaporation ponds) in the world. 13 Extracting lithium from brines requires solar energy to allow for the evaporation of water and large dumpsites are used to store the salt residue generated during the process. 14 This can take up to 24 months.15
Although this method is more sustainable than the conventional mining of lithium, the extraction process in Chile does have a negative impact on the surrounding environment.16 Water is currently the main source of controversy as mining consumes around 65% of water available in the Salar de Atacama region.17The intense use of water in this region not only negatively impacts the surrounding flora and fauna but also the neighbouring communities, whose livelihoods rely on the already scarce supply of water. If the demand for lithium increases, as it has been forecasted to, then these issues will only intensify further.18
Improvements in extraction technology will, in part, improve the sustainability of the first stage in the life cycle of the Li-ion battery (cobalt, nickel and other raw materials used in Li-ion batteries would require similar life cycle analysis to further improve the sustainability). The European Commission has been involved in research that is developing a more innovative and sustainable method of lithium extraction from salt brines.19 The project is known as the Lithium Direct Extraction Process and if successful will not only allow for the industrialisation of high quality lithium, but will also reduce its environmental impact.
The process is an innovative alternative to solar evaporation using a solid to liquid extraction technology. Lithium-depleted brine is returned to the natural environment instead of storing it in large waste sites. In doing so, the impact on the hydric balance will be significantly reduced.20 This process would not only alleviate pressure from areas like the Atacama Desert, it would also enable lithium extraction by countries previously unable due to inadequate natural conditions for effective evaporation, such as a lack of sunshine or lower altitudes.
How rechargeable batteries are made
Once the lithium is extracted, along with the other necessary raw materials, the manufacturing of the Li-ion battery takes place. Li-ion batteries are composed of three layers: an anode, a cathode, and a porous separator.21 The anode is composed of graphite and other conductive additives. The cathode is composed of layered transition metal oxides. The product is saturated in an electrolyte solution, consisting of lithium-salt and organic solvents and sealed in a casing usually composed of steel or aluminium material to create a battery cell.22
Once the battery cell is complete, several cells are arranged to form a battery pack. The battery cells are separated within the battery pack and housed with other components, including a thermal control unit, wiring, and electronic card. Please see Diagram 1 for a more visual description of this process.23
Diagram 1: Life Cycle of a battery. 24 Source: United State Environmental Protection Agency
Waste management
The lifespan of an electric vehicle is projected at being over ten years, yet the lifespan of a Li-ion battery is less than this.25 By the time a Li-ion battery reaches 60%-80% capacity it will reduce the driving range for an EV and will need to be replaced.26 According to a report titled “Circular opportunities in the lithium-ion industry” by Hans Eric Melin, the availability of used Li-ion batteries ready for recycling will exceed 150,000 tonnes by 2025.27 This quantity of battery would generate more than 20,000 tonnes of lithium and 15,000 tonnes of cobalt. Please see Graphs 2 and 3 for a more comprehensive understanding.
Graph 2: Materials from recycled lithium-ion batteries in 2025. Courtesy: Bloomberg Energy Finance
Graph 3: Lithium-ion batteries availability for recycling in 2025. Courtesy: Bloomberg Energy Finance
Technologies that recycle all components of a lithium battery would require the ability to: discharge the spent lithium battery; dismantle and classify the components; separate the electrode components; and refine and add value.28 However, the current reality of the recycling of Li-ion batteries is that although it has the ability to achieve up to 95% recovery, most of the techniques are energy-intensive processes and would require a much higher investment in comparison to the cost of lithium extraction and importation from countries such as Chile.29
Diagram 2: End of life methods. Courtesy: Hong-Chao Zhang
The UK has recently announced that there will be investments in 27 research projects involving 66 organisations for the innovation of battery technology. The projects aim to put the UK on the map as a global leader in the development and manufacturing of new battery technology. In addition, there will be projects that will research and identify more sustainable end uses for batteries by either reusing, remanufacturing or recycling them.30 To this end, the Nissan LEAF factory in Sunderland has also been advocating for the transition to more sustainable lifestyles through technology that reuses EV Li-ion batteries to store solar energy.31 Not only will this create societal access to more sustainable lifestyles, but it also increases the sustainability and lifespan within the life cycle of an Li-ion battery.
Next Steps
The assessment of a product’s life cycle can help pinpoint areas in which the production process can be improved to achieve greater sustainability and reduce environmental damage. New technology could reshape the life cycle of an Li-ion battery and increase its sustainability. In doing so, an electric vehicle’s overall life cycle would expect to have a reduced carbon footprint.
In the future, the recycling of Li-ion batteries could reduce pressure on the extraction of lithium and other raw materials that are necessary in the composition of a battery. In addition, new, innovative extraction techniques will not only relieve the pressure of water supply for local communities in Chile but will also open the lithium market to countries previously hindered by lack of solar energy for the evaporation process. In doing so, the demand for lithium produced in Chile may fluctuate. With this in mind, the government of Chile has been considering both a value-added approach as well as investing in research and technological development to allow for the production of Li-ion batteries.32
Chile, one of the leaders in lithium production, and the UK as a leader in battery innovation and waste management provide a bi-regional perspective of the processes that must be considered when assessing the life cycle of a product. Extant literature promotes an interdisciplinary approach when developing sustainable technologies on an international level.33 In the past, trade agreements between the European Union and Chile have included articles related to the promotion of the exchange of knowledge and experience between the two regions.34 This agreement could lay out the foundation for a new and more tailored model to share science and technology-based knowledge specifically in relation to the lithium-ion battery.
Continued learning between stakeholders within the value chain of the production of electric vehicles and the promotion of innovation, science and technology will further the successes in achieving a sustainable life cycle.
References
1 Shankleman, J. et al. (2018). We’re Going to Need More Lithium. Bloomberg.com (7 Sept.2017). Available at: https://www.bloomberg.com/graphics/2017-lithium-battery-future/ (Accessed 26 Jul. 2018).
2 Shankleman, J. et al. (2018). We’re Going to Need More Lithium. Bloomberg.com (7 Sept.2017). Available at: https://www.bloomberg.com/graphics/2017-lithium-battery-future/ (Accessed 26 Jul. 2018).
3 REDUse, Friends of the Earth, Global 2000 (2013). Less is more Resource efficiency through waste collection, recycling and reuse of aluminium, cotton and lithium in Europe. Brussels: GLOBAL 2000 Verlagsges.m.b.H., Neustiftgasse 36, 1070 Vienna., pp.3-10. Available at: http://www.foeeurope.org/sites/default/files/news/foee_report_-_less_is_more.pdf (Accessed 10 Jun. 2018).
4 Sun, X. et al. (2017). Tracing global lithium flow: A trade-linked material flow analysis. Resources, Conservation and Recycling Research gate, 124(50-61). Available at: https://www.researchgate.net/publication/316747087_Tracing_global_lithium_flow_A_trade-linked_material_flow_analysis. (Accessed 24 Jul. 2018).
5 Zinke, R. et al. (2018). U.S. Geological Survey, Mineral Commodity Summaries, January 2018. Available at:https://doi.org/10.3133/70194932. (Accessed 26 Jul. 2018) 98 p.
6 Sun, X. et al. (2017). Tracing global lithium flow: A trade-linked material flow analysis. Resources, Conservation and Recycling Research gate, 124(50-61). Available at: https://www.researchgate.net/publication/316747087_Tracing_global_lithium_flow_A_trade-linked_material_flow_analysis. (Accessed 24 Jul. 2018).
7 Ibid
8 Sun, X. et al. (2017). Tracing global lithium flow: A trade-linked material flow analysis. Resources, Conservation and Recycling Research gate, 124(50-61). Available at: https://www.researchgate.net/publication/316747087_Tracing_global_lithium_flow_A_trade-linked_material_flow_analysis. (Accessed 24 Jul. 2018).
9 Tahil, W. (2007). The Trouble with Lithium Implications of Future PHEV Production for Lithium Demand. Meridian International Research (January,2007) pp.p.1-p.27. Available at: http://meridian-int-res.com/Projects/Lithium_Problem_2.pdf (Accessed 4 Jun. 2018).
10 Ibid
11Armand, M. and Tarascon, J. (2008). Building better batteries. Nature, 451(7179), pp.652-657. Available at:
https://www.researchgate.net/publication/5594867_Building_Better_Batteries. (Accessed 4 Jun. 2018).
12 Armand, M. and Tarascon, J. (2008). Building better batteries. Nature, 451(7179), pp.652-657. Available at: https://www.researchgate.net/publication/5594867_Building_Better_Batteries (Accessed 4 Jun. 2018).
13 REDUse, Friends of the Earth, Global 2000 (2013). Less is more Resource efficiency through waste collection, recycling and reuse of aluminium, cotton and lithium in Europe. [online] Brussels: GLOBAL 2000 Verlagsges.m.b.H., Neustiftgasse 36, 1070 Vienna., pp.3-10. Available at: http://www.foeeurope.org/sites/default/files/news/foee_report_-_less_is_more.pdf (Accessed 10 Jun. 2018).
14 EUROPEAN COMMISSION (2018). COMMISSION STAFF WORKING DOCUMENT Report on Raw Materials for Battery Applications. Brussels, pp.35-42. Available at: https://ec.europa.eu/transport/sites/transport/files/3rd-mobility-pack/swd20180245.pdf (Accessed 26 Jul. 2018).
15 Ec.europa.eu. (2018). Development of an innovative and sustainable lithium extraction process from medium lithium grade brines – European Commission. Available at: https://ec.europa.eu/growth/tools-databases/eip-raw-materials/en/content/development-innovative-and-sustainable-lithium-extraction-process-medium-lithium-grade (Accessed 26 Jul. 2018).
16 Notter, D. et al. (2010). Contribution of Li-Ion Batteries to the Environmental Impact of Electric Vehicles. Environmental Science & Technology, 44(17), pp.6550-6556. Available at: https://pubs.acs.org/doi/ipdf/10.1021/es903729a (Accessed 26 Jul. 2018).
17 Tahil, W. (2007). The Trouble with Lithium Implications of Future PHEV Production for Lithium Demand. Meridian International Research (January,2007) pp.p.1-p.27. Available at: http://meridian-int-res.com/Projects/Lithium_Problem_2.pdf (Accessed 4 Jun. 2018).
18 USGS (2018). U.S. Geological Survey, Mineral Commodity Summaries. Minerals.usgs.gov. (2018). Available at: https://minerals.usgs.gov/minerals/pubs/commodity/lithium/mcs-2018-lithi.pdf (Accessed 26 Jul. 2018).
19 Ec.europa.eu. (2018). Development of an innovative and sustainable lithium extraction process from medium lithium grade brines – European Commission. Available at: https://ec.europa.eu/growth/tools-databases/eip-raw-materials/en/content/development-innovative-and-sustainable-lithium-extraction-process-medium-lithium-grade (Accessed 26 Jul. 2018).
20 Ec.europa.eu. (2018). Development of an innovative and sustainable lithium extraction process from medium lithium grade brines – European Commission. Available at: https://ec.europa.eu/growth/tools-databases/eip-raw-materials/en/content/development-innovative-and-sustainable-lithium-extraction-process-medium-lithium-grade (Accessed 26 Jul. 2018).
21 Amarakoon, S. et al. (2013). Application of LCA to Nanoscale Technology: Li-ion Batteries for Electric Vehicles. United States Environmental Protection Agency (EPA) National Risk Management Research Laboratory EPA’s Office of Research and Development (2013). Available at: https://www.epa.gov/sites/production/files/201401/documents/lithium_batteries_lca.pdf (Accessed 26 Jul. 2018)
22 Amarakoon, S. et al. (2013). Application of LCA to Nanoscale Technology: Li-ion Batteries for Electric Vehicles. United States Environmental Protection Agency (EPA) National Risk Management Research Laboratory EPA’s Office of Research and Development (2013). Available at: https://www.epa.gov/sites/production/files/201401/documents/lithium_batteries_lca.pdf (Accessed 26 Jul. 2018)
23 Amarakoon, S. et al. (2013). Application of LCA to Nanoscale Technology: Li-ion Batteries for Electric Vehicles. United States Environmental Protection Agency (EPA) National Risk Management Research Laboratory EPA’s Office of Research and Development (2013). Available at: https://www.epa.gov/sites/production/files/201401/documents/lithium_batteries_lca.pdf (Accessed 26 Jul. 2018)
24 Amarakoon, S. et al. (2013). Application of LCA to Nanoscale Technology: Li-ion Batteries for Electric Vehicles. United States Environmental Protection Agency (EPA) National Risk Management Research Laboratory EPA’s Office of Research and Development (2013). Available at: https://www.epa.gov/sites/production/files/201401/documents/lithium_batteries_lca.pdf (Accessed 26 Jul. 2018)
25 Vikström, H. et al . (2013). Lithium availability and future production outlooks. Science Direct 110, pp.252-266. Available at: https://doi.org/10.1016/j.apenergy.2013.04.005 (Accessed 26 Jul. 2018).
26 Ethan N. Elkind (2014). REUSE AND REPOWER How to Save Money and Clean the Grid with Second-Life Electric Vehicle Batteries. UCLA School of Law’s Emmett Institute on Climate Change and the Environment and UC Berkeley School of Law’s Center for Law, Energy & the Environment. (2018). Available at: https://www.law.berkeley.edu/files/ccelp/Reuse_and_Repower_–_Web_Copy.pdf (Accessed 26 Jul. 2018).
27 Melin, H. (2018). Press release: Recycled lithium to reach 9 per cent of total lithium battery supply in 2025. Circular Energy Storage(2018). Available at: https://circularenergystorage.com/news/2017/11/30/press-release-recycled-lithium-to-reach-9-percent-of-total-lithium-battery-supply-in-2025 (Accessed 26 Jul. 2018).
28 Lebedeva, N. et al. (2016). Lithium ion battery value chain and related opportunities for Europe. European Commission.(2016) Available at: https://ec.europa.eu/jrc/sites/jrcsh/files/jrc105010_161214_li-ion_battery_value_chain_jrc105010.pdf (Accessed 26 Jul. 2018).
29 Lebedeva, N. et al. (2016). Lithium ion battery value chain and related opportunities for Europe. European Commission (2016) Available at: https://ec.europa.eu/jrc/sites/jrcsh/files/jrc105010_161214_li-ion_battery_value_chain_jrc105010.pdf (Accessed 26 Jul. 2018).
30 GOV.UK (2017). Future electric vehicle batteries: long-lasting, cleaner, better. GOV.UK (2017). Available at: https://www.gov.uk/government/news/future-electric-vehicle-batteries-long-lasting-cleaner-better#history (Accessed 26 Jul. 2018).
31 Nissan. (2018). Clean power energy | Nissan. xStorage by Nissan(2018) Available at: https://www.nissan.co.uk/experience-nissan/electric-vehicle-leadership/xstorage-by-nissan.html (Accessed 26 Jul. 2018).
32 Sophia Boddenberg (2018). Chile’s lithium – blessing or curse? Made for minds(2018). Available at: https://www.dw.com/en/chiles-lithium-blessing-or-curse/a-43721539 (Accessed 26 Jul. 2018).
33 Tarascon, J. (2018). Key challenges in future Li-battery research. Philosophical Transaction of the Royal Society a Mathematical, Physical and Engineering Science(2018). Available at: https://p.dw.com/p/2xRy7(Accessed 26 Jul. 2018).
34 European Commission (2002). EU – Chile Association Agreement. European Commission (2002). Available at: https://eur-lex.europa.eu/resource.html?uri=cellar:f83a503c-fa20-4b3a-9535-f1074175eaf0.0004.02/DOC_2&format=PDF (Accessed 15 Aug.2018).
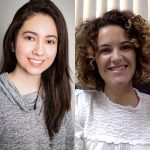
Eleanor Gibson (Scotland) and Scarleth Vasconcelos (Nicaragua)
Eleanor Gibson holds an International Masters in Sustainable Business and Innovation from EADA Business School in Barcelona and a Bachelor in Geography and Spanish from the University of Glasgow. Her interests lie in the field of environmental studies, sustainable development and education. She worked at the Guyana Green State Development Strategy Coordination Office toward countrywide sustainable development and economic growth.She also has experience carrying out volunteer work in Cameroon, where she helped develop a social project for the sustainable use of water, and has spent six months living in Panama working as an intern in green economy and resource efficiency for UN Environment. Scarleth Vasconcelos holds a Master in Sustainable Engineering from Villanoca University, U.S.A, and a Bachelor’s degree in Renewable Energy Engineering in Nicaragua. She gained practical experience researching for the Electrical and Computer Engineering department at Villanova University. During her bachelor studies, she volunteered projects related to renewable energy (photovoltaic systems) and ecofriendly stoves in rural communities in Nicaragua. Scarleth was also a volunteer at UNICEF, Nicaragua where she and her team participated in “The Global Design for UNICEF ” 2014, proposing a telehealth project for communities in the Carribean Coast of Nicaragua and winning the first place worldwide of the contest.